Introduction
- The chemical bond formation was first interpreted by the Lewis approach. However, the origin of covalent bonds and the nature of the attraction force between neighbouring atoms in molecules remain unexplained.
- As a solution, German scientists Fritz Wolfgang London and Walter Heinrich Heitler developed the valence bond hypothesis. Schrodinger’s wave equation was used to characterise the formation of a covalent bond between two hydrogen atoms by electron sharing.
- The principles of electronic configuration, electrical structure and atomic orbitals (and their overlap), and the hybridisation of most atomic orbitals are the main topics of this theory. Atomic orbitals that overlap and the electrons that are concentrated in the matching bond area create chemical bonds.
What is Valence Bond Theory?
The valence bond theory describes the electrical structure of atoms and molecules. The concept explains how electrons are distributed among a molecule’s atomic orbitals. To better understand chemical bonding, the VBT incorporates quantum mechanical principles and explains the electrical makeup of molecules.
According to this theory, all the potential energy levels of an atom in a molecule are occupied, and all bonds are localized, atomic-scale bonds between two atoms that involve sharing an electron by both atoms. Because each atom has only one unpaired electron, their orbitals are weakly connected.
The two atomic orbitals don’t need to be the same. There can be interactions between, say, s and p orbitals. A sigma bond is formed when the orbitals of the two shared electrons intersect. However, Pi bonds are formed when the orbitals cross but remain perpendicular to one another. Since these atomic orbitals will overlap, the possibility of an electron existing in the bond position is highest. Because of the overlapping, electrons are most likely in the bond region.
Postulates of Valence Bond Theory
The main postulates of the Valence Bond Theory are outlined below:
- When two or more atoms come together, their unfilled outer electron shells overlap, creating a covalent bond.
- The orbitals of atoms must be sufficiently near together and properly oriented for overlap to occur.
- The only way for atoms to bind is through sharing electrons, which occurs when their valence orbitals overlap.
- There is an electron pair in overlapping orbitals, and their spins are antiparallel to one another.
- How tightly the orbitals overlap directly affects the quality of the established bond. Stronger covalent bonds are created when more atomic orbitals overlap.
- When atoms combine to form a molecule, the resulting structure is identical to the original atoms.
Need for Valence Bond Theory
Combining the ideas of Lewis’s pair bonding with the Heitler-London theory, Linus Pauling proposed the valence bond theory in 1928. The concept of the valence bond was developed to account for resonance and orbital hybridisation.
Written by Pauling and published in 1931, “On the Nature of the Chemical Bond” is an in-depth analysis of valence bonds.
Lewis provides the systems to explain how molecules are arranged. However, it omitted to discuss the formation of chemical bonds. Similarly to that, the VSEPR hypothesis defines how essential molecules seem. However, its scope of use was relatively constrained. Additionally, the geometry of a complex atom was not described. To address and resolve these restrictions, scientists had to introduce the notion of valence bonding.
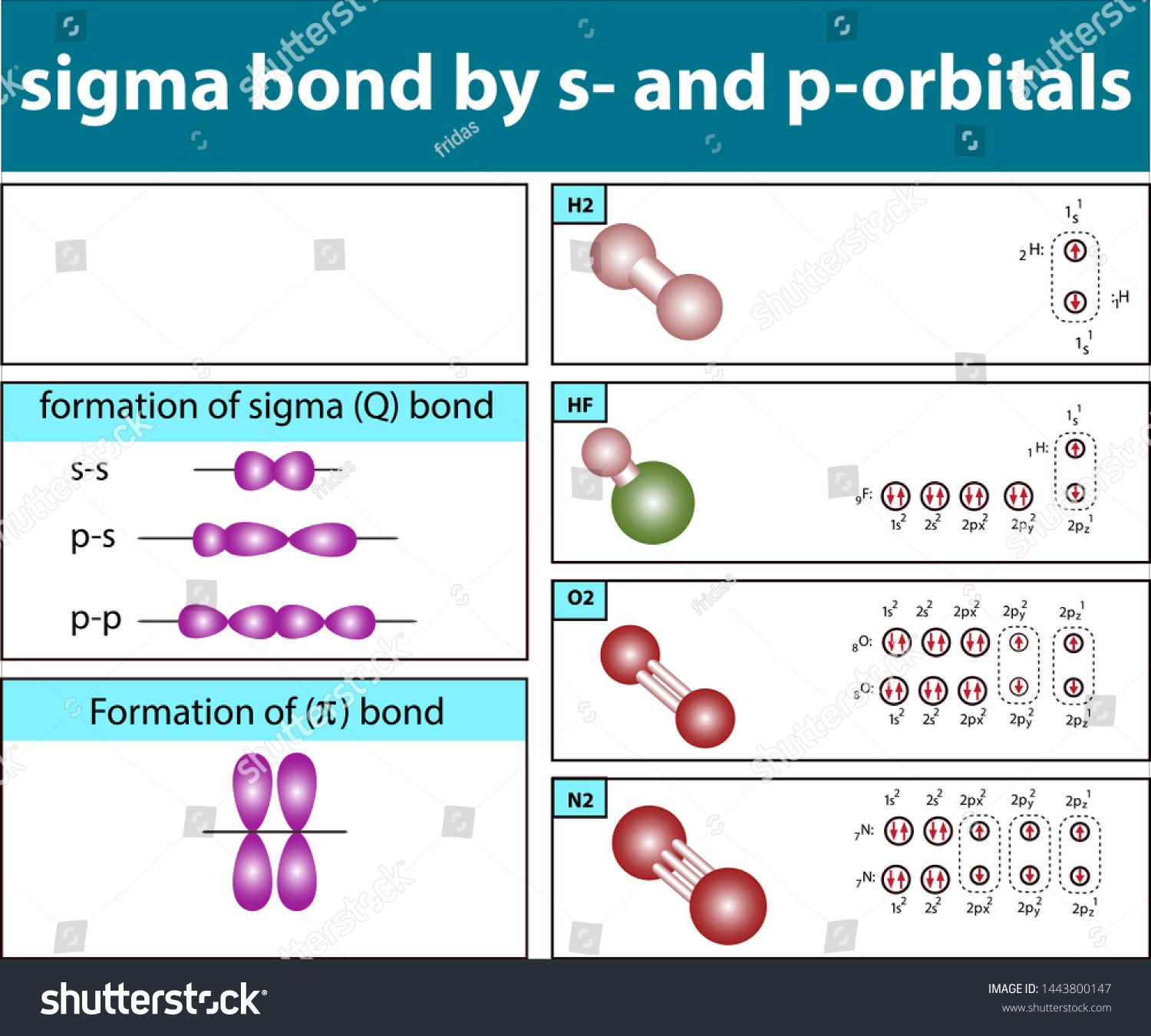
Modern Valence Bond Theory
- As an alternative to the valence bond idea, in which electron pairs are thought to be situated between a molecule’s two distinct atoms, covalent bonds can exist between atoms.
- Molecular orbital theory is the backbone of contemporary valence bond theory, which proposes that electron pairs are distributed around the molecule in sets of molecular orbitals.
- Predictions of magnetic and ionisation properties are simplified by molecular orbital theory, but valence bond theory provides greater nuance.
- The aromatic properties of molecules are attributed to the spin coupling between the pi orbitals, as proposed by contemporary valence bond theory.
- There is not much difference between the Dewar and Kekule arrangements from the perspective of the concept of resonance.
- According to molecular orbital theory, aromaticity is conceptualised as the delocalisation of the pi-electrons. According to molecular orbital theory, the ions participating in a chemical reaction are incorrectly predicted by the function representing the hydrogen molecule. In turn, this causes atoms to link together chemically.
Applications Of Valence Bond Theory
- VBT’s most significant use is in predicting molecular structure. VBT can be used to predict the shape and size of molecules by taking into account the distribution of electrons among atoms.
- The formation of chemical bonds can also be understood using VBT. VBT can explain the type of bond formed between two atoms by understanding the electron-sharing between the atoms. Predicting the strength of a bond and the reactivity of a molecule are two areas where this is particularly helpful.
- The physical and chemical properties of a molecule, like its melting and boiling points, can be predicted with this method.
Summary
The valence bond theory is a chemical bonding concept that describes the nature of the chemical bond between two atoms. Like molecular orbital (MO) theory, it employs quantum mechanical principles to describe bonding. According to the valence bond theory, bonds are formed when unoccupied atomic orbitals overlap. To form a bond, the two atoms use the same unpaired electron to occupy an orbital, resulting in a hybrid orbital. Both sigma and pi bonds are accounted for in the valence bond theory.
Frequently Asked Questions
1. What are the limitations of the Valence Bond Theory?
Ans: Valence Bond Theory is limited in its ability to explain the behaviour of electrons in molecules that contain more than two atoms. It is also limited in its ability to explain the behaviour of electrons in molecules that contain multiple bonds.
2. How does the Molecular Orbital Theory vary from the Valence Bond Theory?
Ans. The Valence Bond Theory explains how atoms in a molecule share electrons to form chemical bonds. Molecular Orbital Theory is based on the idea that electrons in a molecule occupy discrete energy levels, or “orbitals,” around the nucleus.
3. To what extent does geometry factor into the theory of valence bonds?
Ans. Quite simply said, valence bond theory relies heavily on geometric considerations. How electron pairs are arranged around a molecule’s core atom determines the structure’s three-dimensional shape. Linear, trigonal planar, tetrahedral, and octahedral arrangements are all possible for the electron pairs. Chemical bond strength and reactivity are both affected by the shape of a molecule, which is determined by the arrangement of electron pairs.